Chemistry in space
Posted: December 20, 2024
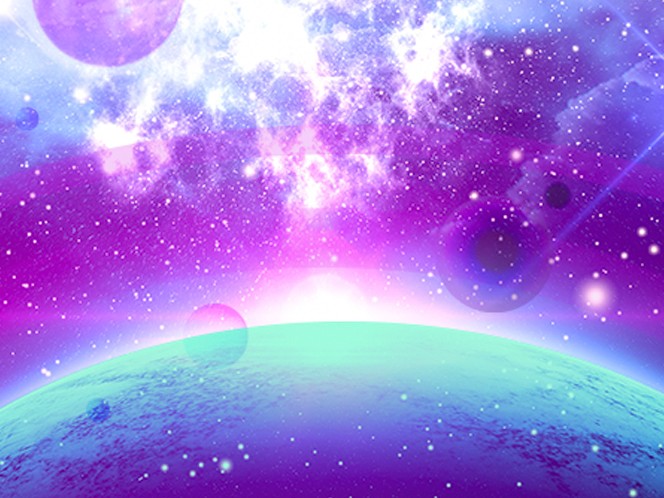
When we gaze at the night sky, far from the city lights, the celestial vault appears as an empty space dotted with stars, as if there is nothing between them. But scientists have discovered that the expanse of interstellar space is actually a gigantic chemistry lab where complex organic molecules manage to form.
So far, hundreds of alcohols, aldehydes, acids, amides, and many other organic compounds have been detected in space. Some are familiar to us on Earth, while others are too unstable to exist here.
The insights gained from space chemistry have far-reaching implications for understanding the origins of life and the universe, and could even improve technologies here on Earth.
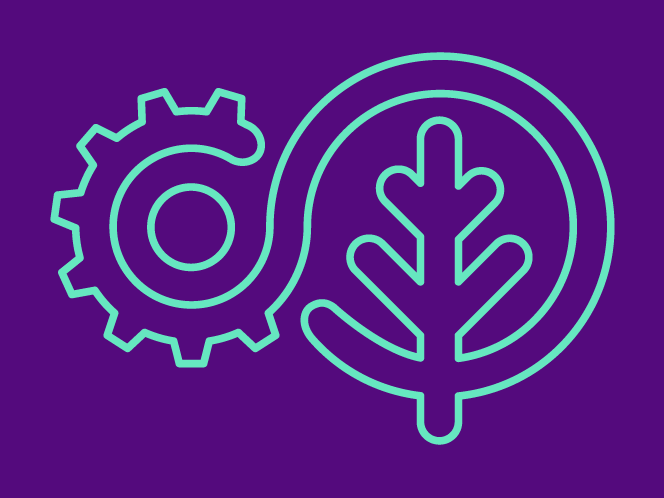
Our Industrial Life
Get your bi-weekly newsletter sharing fresh perspectives on complicated issues, new technology, and open questions shaping our industrial world.
Cosmic chemistry: Complex molecules can form in space
For decades, scientists believed the harsh conditions of space made the formation of complex carbon-based molecules impossible. However, discoveries over the past century have revealed space as surprisingly hospitable to molecular complexity. Early detections of simple molecules like hydrogen and nitrogen evolved into findings of water, ethanol, and formaldehyde in molecular clouds. These molecules, some essential precursors to organic compounds, demonstrated that the building blocks of life could form even in extreme environments.
A significant breakthrough occurred in 2018 when the Green Bank Telescope in West Virginia focused on the Taurus Molecular Cloud 1 (TMC-1) detected cyanobenzene, a carbon-based ring structure. This was the first unambiguous detection of a specific aromatic molecule in interstellar space.[1][2]
Around the same time, scientists analyzing light from TMC-1 identified polycyclic aromatic hydrocarbons, indicating that aromatic rings can form and persist even under the cold and low-density conditions typical of molecular clouds.
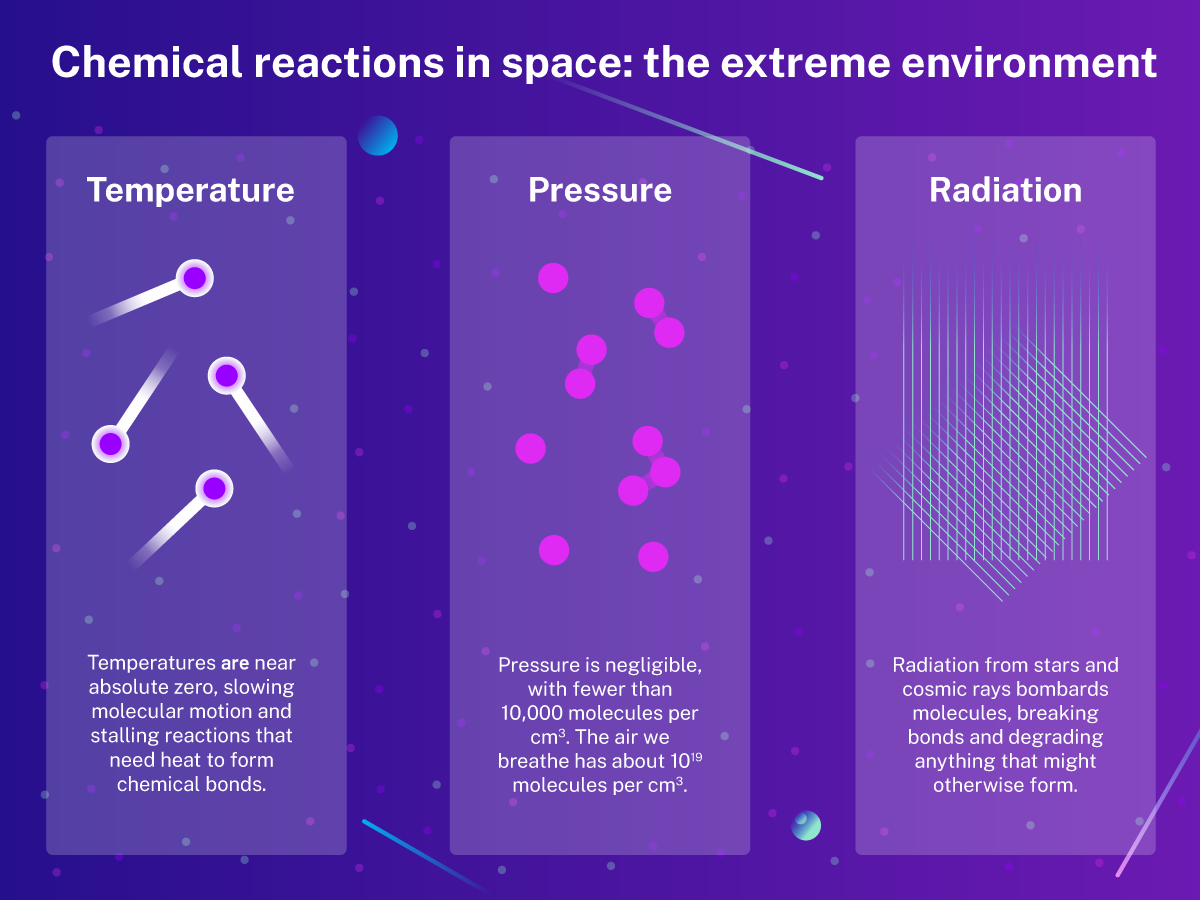
The discovery of complex organic rings in space has profound implications for our understanding of the universe and the origins of life. Polycyclic aromatic hydrocarbons and other aromatic molecules in interstellar environments are precursors to biologically important compounds such as DNA, peptides, and proteins.
In a recent study, researchers hypothesized that peptides could have formed in nascent solar systems, a theory that could provide an intriguing alternative pathway for understanding how life could have emerged on Earth.
Their research focuses on the dense environments of dusty disks from which new solar systems are born. These disks form when clouds collapse under gravitational forces, creating conditions where water molecules become prevalent and form ice on the surfaces of growing particle agglomerates, inhibiting the reactions that form peptides.
As rocks and dust coalesce into larger bodies like asteroids and comets, the resulting heat allows liquid water to form, boosting peptide synthesis and leading to a natural selection of further reactions that produce even more complex organic molecules. Such processes could have happened during the formation of our own Solar System.
The ability of these complex molecules to form in the harsh conditions of space increases the likelihood that life, or at least the precursors to life, might exist elsewhere in the universe.
Microgravity and the potential of space chemistry
Microgravity, or zero gravity, is a condition in which gravitational forces are drastically reduced to about one-millionth of Earth's gravity (10⁻⁶ g). This unique environment profoundly influences chemical reactions, offering both challenges and opportunities for scientific exploration.
One of the most significant effects of microgravity is the elimination of gravity-driven phenomena such as convection and sedimentation. On Earth, convection currents efficiently mix reactants as warmer, less dense fluids rise and cooler, denser fluids sink. At the same time, sedimentation allows heavier particles to settle in mixtures.
In microgravity, these processes vanish, leaving diffusion—the random movement of molecules—as the primary mixing mechanism. While diffusion is slower and less efficient, it creates conditions for producing materials with exceptional uniformity and precision.
Microgravity enables the creation of materials with highly uniform microstructures.[3] Without density-driven gradients during solidification, materials such as semiconductors and metal foams achieve more homogeneous compositions, improving their physical and chemical properties.
For example, semiconductors and metal alloys fabricated in microgravity have fewer defects and more homogeneous microstructures. This leads to improved electrical conductivity, mechanical strength, and thermal performance—qualities essential for high-tech devices such as smartphones, solar panels, and advanced aerospace equipment. NASA’s researchers aim to eliminate the need for etching in semiconductor manufacturing by utilizing the reduced pressure in space to create thinner, more efficient semiconductor layers.
Microgravity allows for the growth of larger, more well-ordered protein crystals compared to those grown on Earth. Experiments on the International Space Station (ISS) have demonstrated that microgravity enables scientists to create purer, more structurally perfect crystals of proteins crucial for determining the detailed structures of proteins, which is essential for drug development.
Metal foams—lightweight materials with a high strength-to-weight ratio—can be produced with greater uniformity in microgravity. These foams have potential applications in aerospace engineering, where reducing weight while maintaining strength is critical for fuel efficiency in spacecraft and aircraft.
Unique microgravity laboratories, such as those on the ISS, provide an unparalleled opportunity for scientists to explore the intricacies of space chemistry. Studying chemistry in this orbital laboratory helps unravel the mysteries of molecular behavior in extreme conditions, offering insights into fundamental chemical principles but also open to potential applications in astrobiology and materials science, and ultimately deepens our knowledge of the universe and the origins of life.
References
[1] Detection of interstellar H2CCCHC3N A possible link between chains and rings in cold cores, Astronomy & Astrophysics, March 2021.
[2] Detection of the aromatic molecule benzonitrile (c-C6H5CN) in the interstellar medium, Science, January 2018.
[3] Science in microgravity, Chemistry World, December 2028.