Nobel-Prize physics relies on industrial data
Posted: September 09, 2024
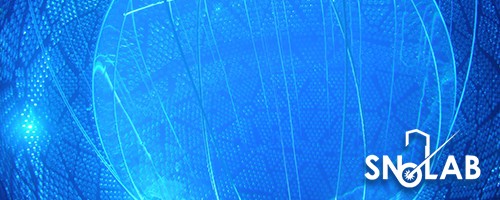
How do you find the lightest, most elusive particles in the universe? You need extremely precise data. And, sometimes you have to go deep underground into a nickel mine in Ontario, Canada. That’s where SNOLAB, the deepest clean lab in the world, conducts cutting-edge physics under 2 km of Canadian Shield rock. That 2 km of rock shields SNOLAB from cosmic rays and radioactivity that could interfere with its delicate experiments. It’s also an ISO six cleanroom, which ensures that scientists don’t inadvertently contaminate their own experiments.
SNOLAB needs to be so deep and so clean because it’s looking for neutrinos: subatomic particles so light and so tiny that we can’t even measure their mass. In fact, scientists used to think that neutrinos didn’t have any mass at all until SNOLAB’s original experiment, SNO, the Sudbury Neutrino Observatory, conducted Nobel-Prize winning research proving that they do.
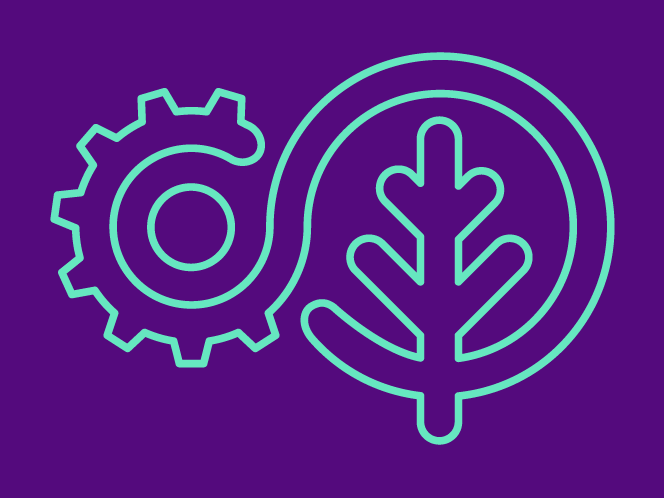
Our Industrial Life
Get your bi-weekly newsletter sharing fresh perspectives on complicated issues, new technology, and open questions shaping our industrial world.
Searching for a grain of sand in the desert
The SNO experiment proved these tiny particles have mass by precisely counting the number of neutrinos streaming from the sun to Earth. The sun generates heat through nuclear fusion, turning hydrogen into helium. For each new helium nucleus the sun creates, it also creates two neutrinos, which stream out from the sun. Hundreds of billions of neutrinos from the sun stream through your body every second. But, they don’t interact with light or any electromagnetic force at all, and very rarely interact with any kind of matter. You only have a 25% chance of a neutrino interacting with your body in the course of your life, even though there are hundreds of trillions zipping through you every second when you add neutrinos from other sources to the hundreds of billions coming from the sun.
So, it’s extremely difficult to detect the neutrinos streaming from the sun. But, if you can accurately detect the few times one of them does interact with a bit of matter, you can extrapolate how many there are in total. Starting in the 1960s, the physicist Raymond Davis Jr. set out to do just that.
In 1965, the Chicago Bridge and Iron Company—known for constructing things like municipal water tanks, reactor containment vessels, and space simulation chambers—built a tank that would eventually hold 390,000 liters of drycleaning fluid and be buried 1.5 km underground in the Homestake gold mine in North Dakota. Davis calculated that every month, about 20 of the neutrinos streaming from the sun should each interact with an atom of chlorine in the drycleaning solution and turn it into an atom of argon.
From 1970 until 1994, he and his team counted the number of argon atoms produced in the Homestake mine. At the end of 24 years, he had counted about 2,000 atoms and verified that he hadn’t missed any, a Nobel Prize-winning achievement described on the Nobel Prize website as “considerably more difficult than finding a particular grain of sand in the whole of the Sahara desert.” His work indicated that about 2,000 neutrinos had interacted with the drycleaning solution in the mine during the 24 years the experiment ran.
The solar neutrino problem
But there was a problem. Those 2,000 neutrinos were only about one third the number scientists had predicted should be detected. So, something had to be wrong with their theory. Either the Sun wasn’t producing as many neutrinos as we thought, or the neutrinos were behaving differently than expected.
SNOLAB went online in 1999 in an attempt to solve this “solar neutrino problem.” Instead of a tank of drycleaning fluid, SNOLAB engineered an acrylic sphere filled with 1,000 tonnes of heavy water—water composed of hydrogen that has an extra neutron in its nucleus. The sphere was surrounded by 9,500 light detectors, which would detect light given off when a neutrino travelling from the sun interacted with the heavy water.
The Homestake mine could only detect one variety of neutrino—the electron neutrino. But, neutrinos come in three different varieties, or “flavors”: electron neutrinos, muon neutrinos and tau neutrinos. SNOLAB’s heavy water could detect both the total number of neutrinos of any flavor as well as the number of just the electron neutrinos. The sun only produces electron neutrinos, so, in theory, the total number of neutrinos coming from the sun detected by SNOLAB should have been equal to the number of electron neutrinos it detected.
But, SNOLAB’s data showed something else. Just like Davis’ experiment, it only found one third the number of electron neutrinos the scientists expected to find. But, in an unexpected twist, the data showed that total number of neutrinos of any flavor precisely matched the number of electron neutrinos predicted to be coming from the sun.
These results, along with others from Dr. Takaati Kajita’s Super-Kamiokande detector in a zinc mine in Tokyo, suggested that the sun was producing electron neutrinos, but some of them were turning into muon or tau nutrinos before they interacted with the neutrino detectors on earth.
The upshot of this discovery that neutrinos oscillate between different flavors is that they must have at least some mass, due to the rather complicated dynamics of quantum physics. In 2015, Arthur B. McDonald from SNOLAB and Takaati Kajita jointly won the Nobel Prize in physics for this discovery.
Experiments at SNOLAB continue to probe the mysterious nature of neutrinos while also searching for dark matter, another substance that’s theorized to exist but difficult to study because, like neutrinos, it doesn’t interact with electromagnetism. Recently, I got to sit down with Steven Back, the Technical Services Manager at SNOLAB, to discuss how good industrial data management makes these sensitive experiments possible in SNOLAB’s hyper-clean conditions deep within the earth.